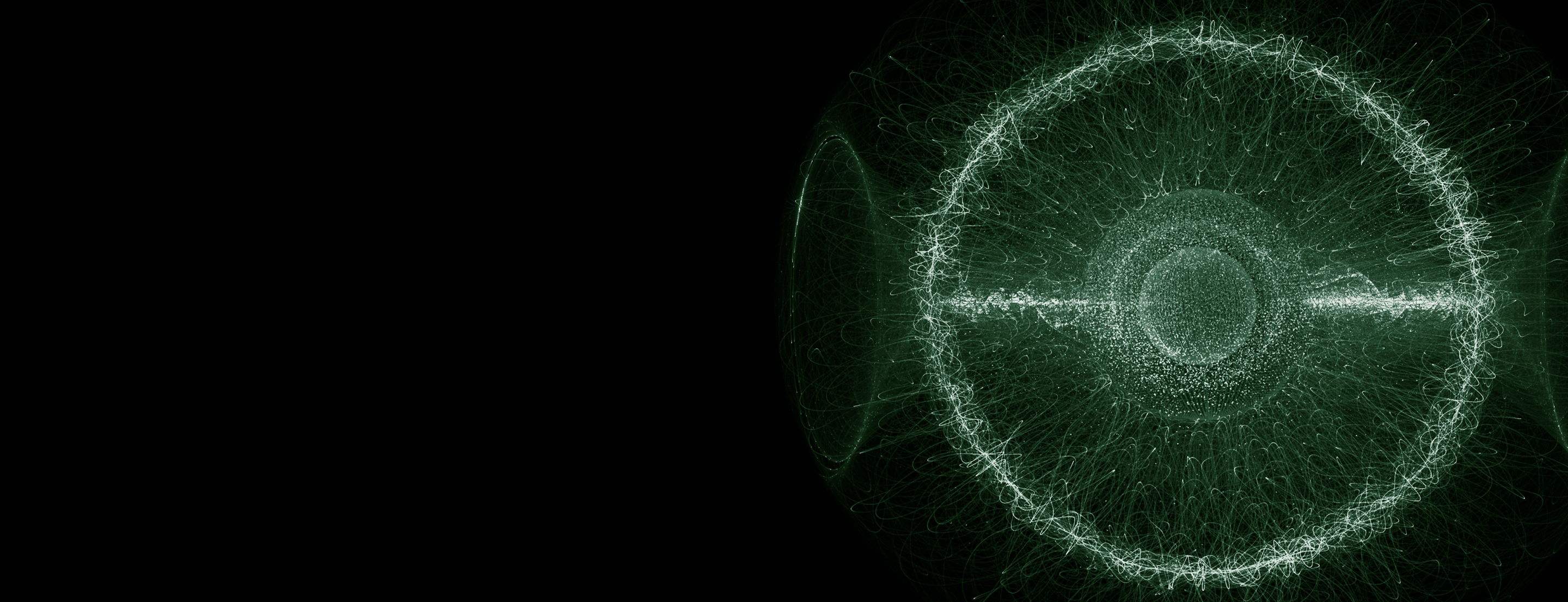
Fusion reactor
Everything you need to know about fusion reactor
What is a nuclear fusion reactor ?
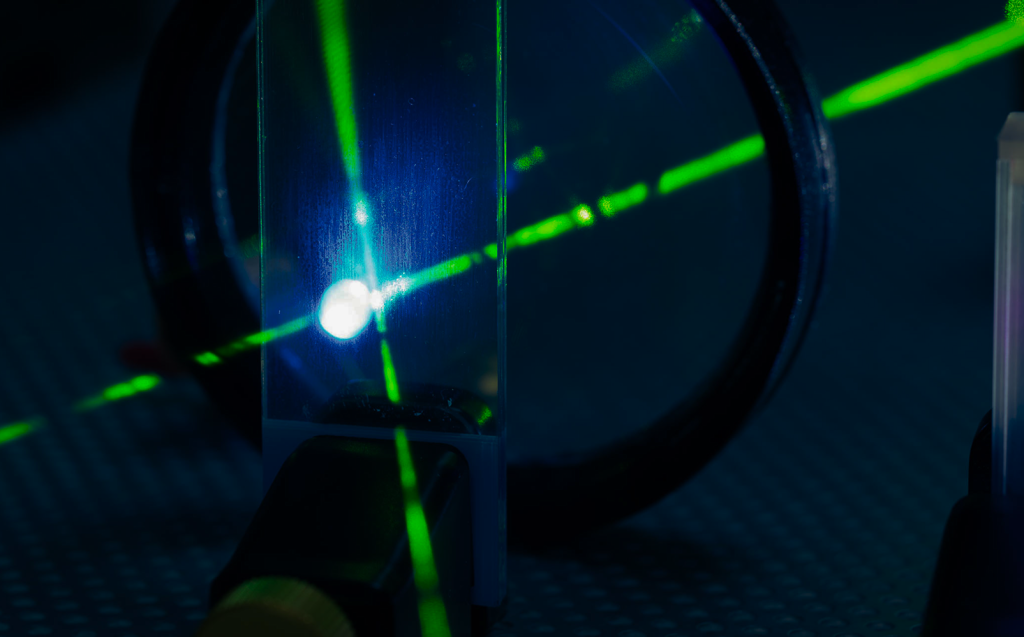
A nuclear fusion reactor is a special type of machine designed to create energy by fusing, or combining, small atoms together. This is different from a fission reactor, which generates energy by splitting large atoms apart.
The fusion reactor prospect landscape includes but is not limited to the following technologies:
- Inertial fusion reactor,
- Magnetic fusion reactor (tokamak, stellarator, WHAM, …) ,
- Magnetic inertial fusion reactor,
- Z-pinch reactor.
No matter the type, all fusion reactor mechanisms operate in a similar way. The goal is to heat up tiny atoms (like hydrogen) so much that they turn into a very hot gas called plasma. The plasma needs to reach temperatures above 100 million degrees Celsius (hotter than the Sun!) and be under high pressure. When this happens, the atoms collide and fuse together, releasing a huge amount of energy—more than what was needed to make them fuse!
At GenF, we focus on Inertial Fusion Reactor (IFR). We chose this approach because of exciting progress made at the National Ignition Facility (NIF) in the United States. Between 2022 and 2024, NIF successfully achieved fusion where the energy released was greater than the energy used to trigger the reaction—this is called a fusion gain greater than one. However, this is different from an engineering gain, which considers the total energy needed to run the whole reactor system. NIF was built mainly for defense research, so its goal was not to produce more energy than it consumes.
How works the Inertial Fusion Reactor ?
Core principle
In Inertial Confinement Fusion (ICF), we use a tiny fuel pellet made of hydrogen isotopes deuterium (D) and tritium (T). This pellet is frozen (cryogenic) and contains both solid and gas states of fuel. The trick is to compress the pellet super-fast using powerful energy sources, trapping the energy inside long enough for fusion to happen. For ICF, what we call long enough is about 10 nanoseconds (10 billion times shorter than a second is).
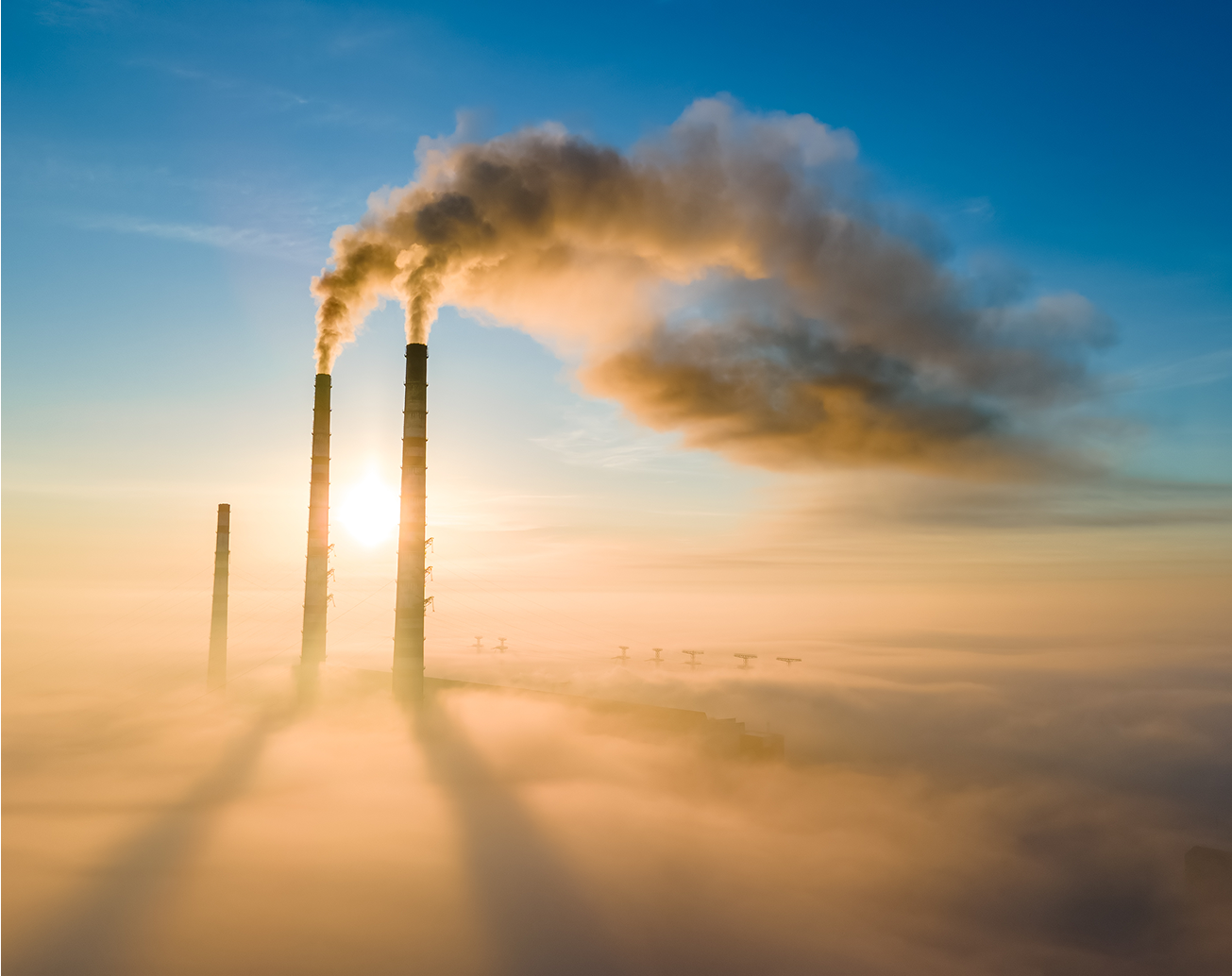
Laser driven compression
To make fusion work, we hit the tiny fuel pellet with hundreds of high-power laser beams inside a large reaction chamber (over 5 meters in radius). These lasers deliver an energy pulse lasting just a few nanoseconds, with energy levels ranging from 0.5 to 5 megajoules (MJ). This rapid energy burst compresses the fuel pellet, making it hot and dense enough for fusion to start.
For this to work properly, the laser beams must hit the pellet evenly from all sides. If the compression is not uniform, the pellet will not ignite correctly.
This is a major engineering challenge because:
- The fuel pellets must be perfectly manufactured,
- The reactor must fire 5 to 20 times per second to be cost-effective.
From fusion to electric energy
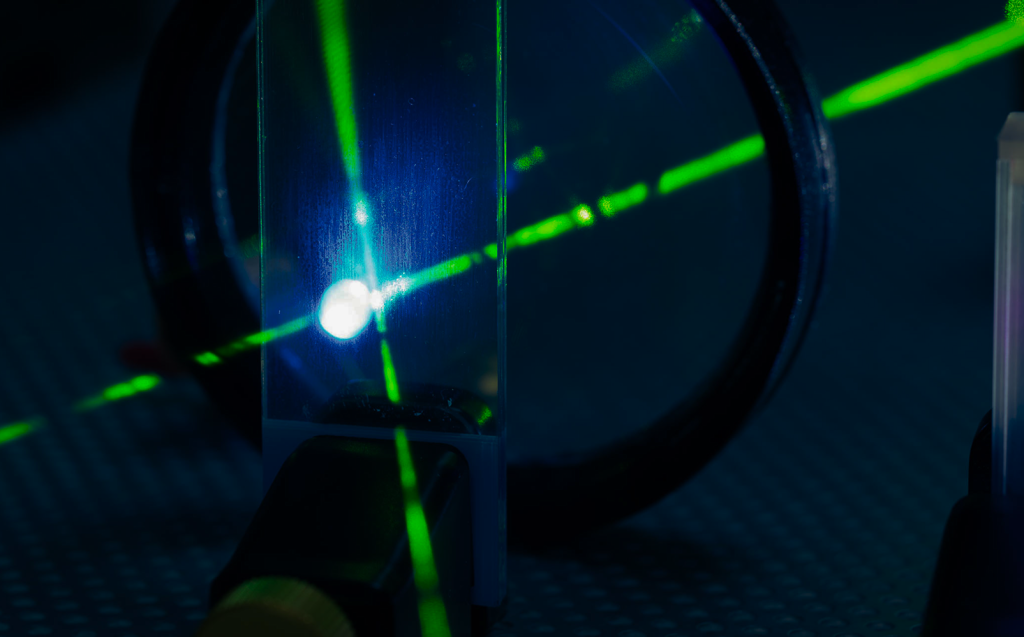
The fusion reaction produces mainly: Neutrons (75%), Ions (23%), X-rays (2%). There are two ways to turn this energy into electricity:
- The reactor has a special wall (called a blanket) that absorbs neutrons, heating up in the process.
- A cooling system transfers this heat to a steam generator.
- The steam drives a turbine, which powers an alternator to produce electricity.
- This method is common but only about 40% efficient.
- Since ions (charged particles) carry energy, special methods can directly capture them to convert energy more efficiently.
An example of a diagram of a fusion reactor with indirect conversion is shown below.
Diagram of an inertial fusion reactor
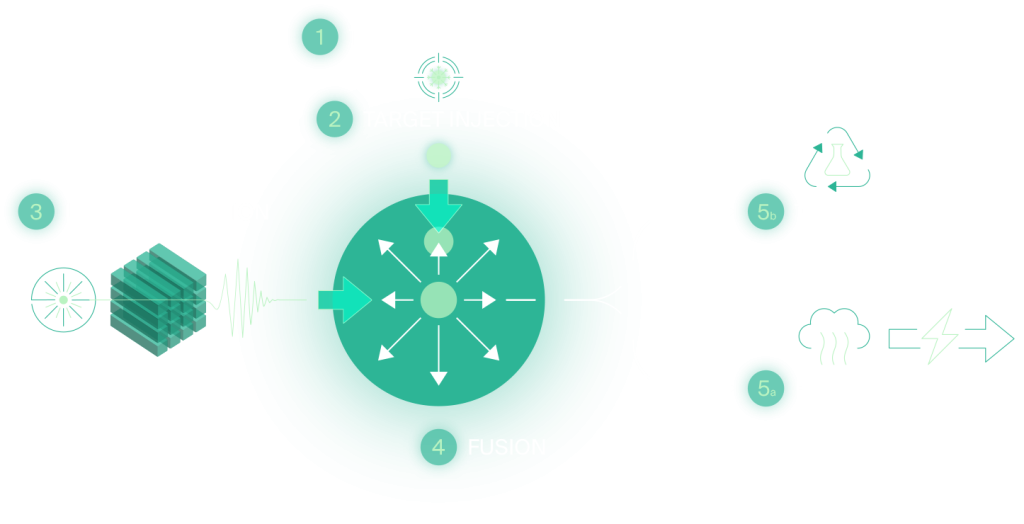
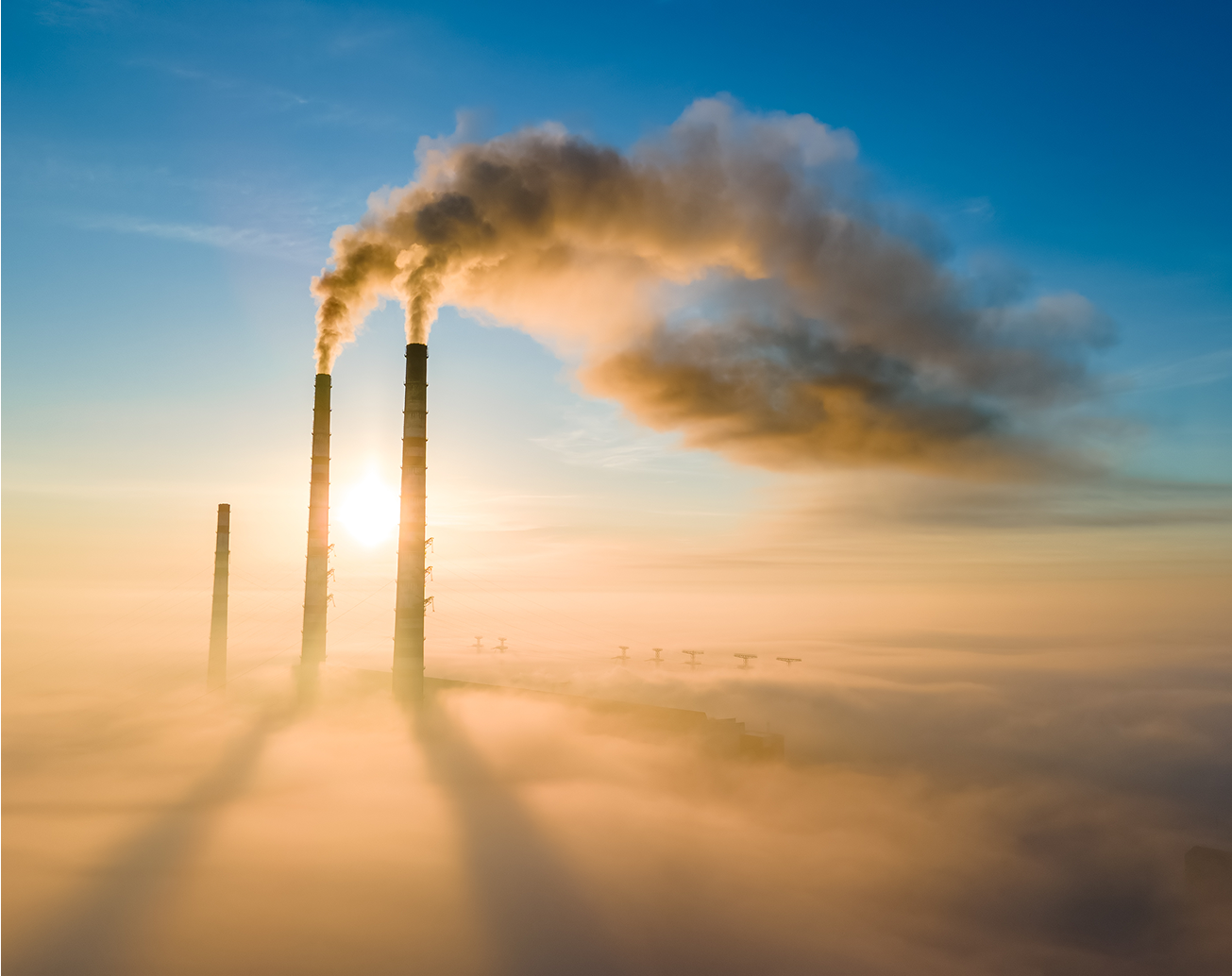
Advantages of an Inertial Fusion Reactor
An Inertial Fusion Reactor has several key advantages:
- Highly controllable: If we stop injecting fuel or firing lasers, the fusion reaction stops immediately.
- Safe operation: There is no risk of a runaway reaction. If something goes wrong with the lasers or fuel injection, fusion simply will not happen—making IFRs very safe.
- Minimal radioactive waste: The only radioactive material involved is tritium, which has a short half-life of about 12 years. Tritium is produced in small amounts and can be contained and recycled within the reactor.
- Fuel self-sufficiency: Every fusion reactor using tritium must generate its own fuel to operate long-term.
A self-sufficient fusion reactor
For an IFR to run efficiently, it needs to be self-sufficient in both fuel production and energy generation.
Fusion Reactor Power Management and Electrical Self-Sufficiency
A key challenge in fusion energy is ensuring that the reactor generates more power than it consumes. By using fusion reactor power management, we can operate a fusion reactor in a close loop, meaning it generates more electricity than it needs to operate. This is crucial for making fusion a viable power source for the future.
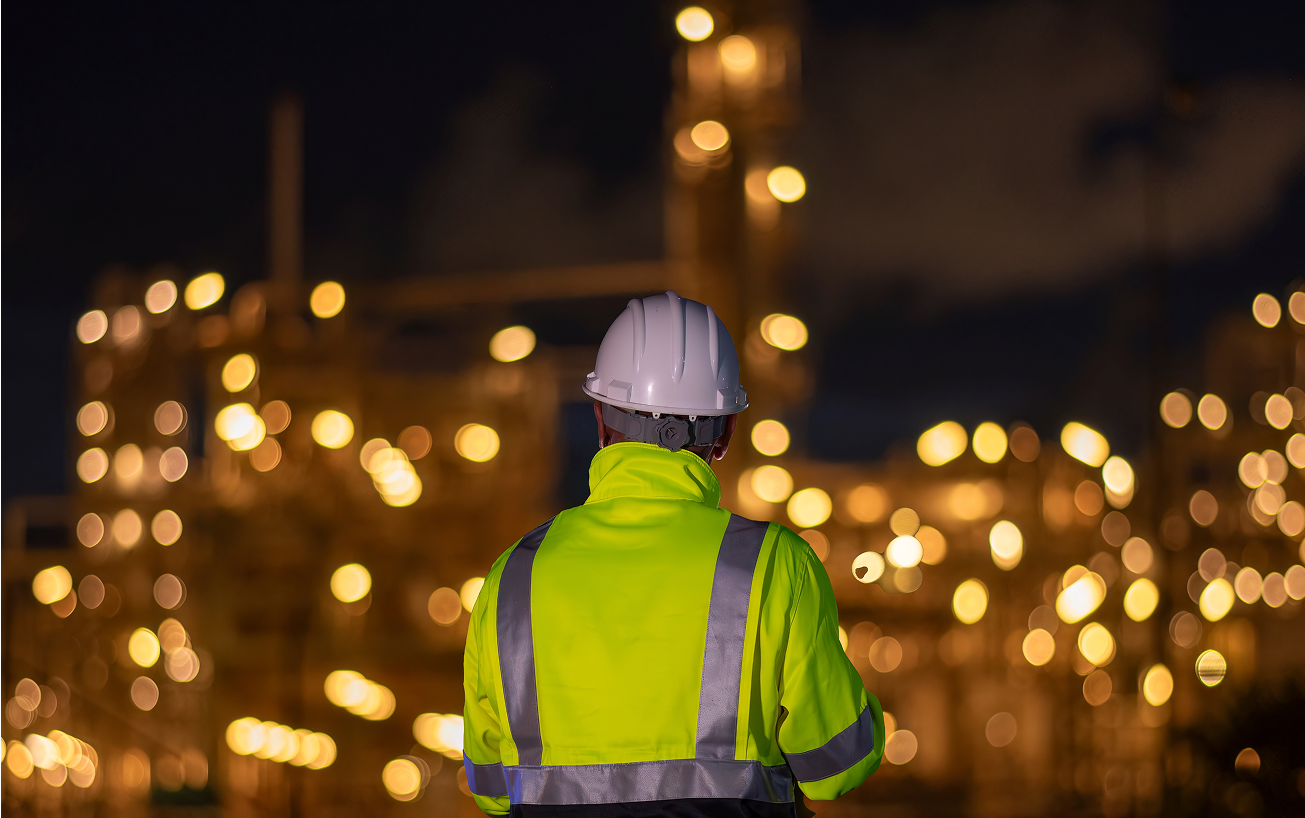
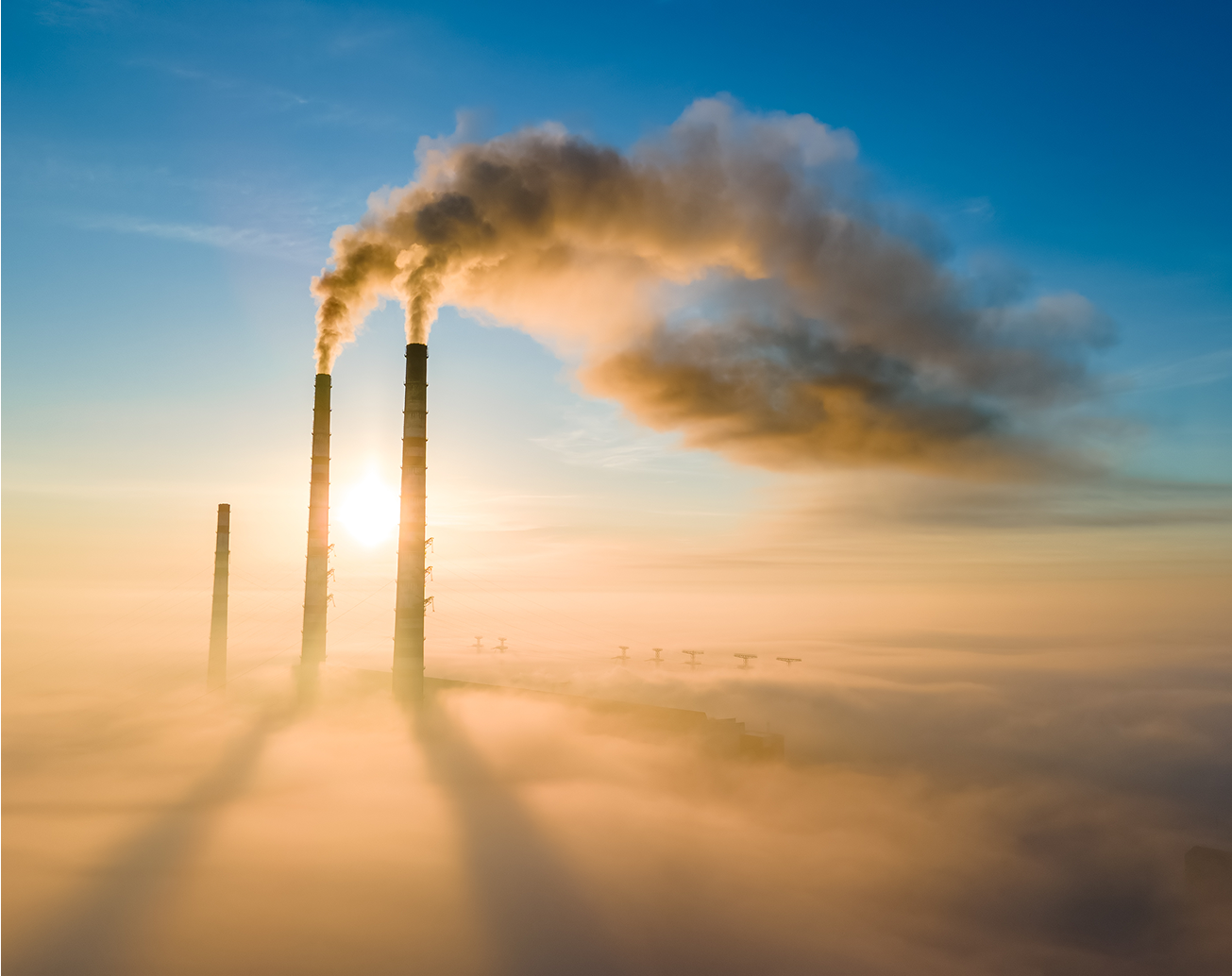
Producing its own fuel
Unlike traditional power plants that simply burn fuel, an IFR must create its own tritium—a rare element on Earth. In 2018, only 35 kg of tritium existed worldwide, and global production was just 2.5 kg per year. However, a fusion reactor, which generates 1 GW of thermal energy (~ 400MW of electric energy), needs about 50 kg of tritium per year—much more than what is currently available.
To solve this, fusion reactors use a breeding blanket, which surrounds the reaction chamber. This blanket contains lithium, which absorbs neutrons from the fusion reaction and transforms into helium and tritium. The tritium is then extracted and reused as fuel.
For a fusion reactor to be truly self-sufficient it must create more tritium than it consumes.
The future of fusion Energy by GenF
Fusion power is one of the most promising ways to generate clean, limitless energy in the future. However, there are still challenges in making it practical and efficient. At GenF, we are working to overcome these challenges and make fusion energy a reality!
At GenF, we focus on Inertial Fusion Reactor (IFR). We chose this approach because of exciting progress made at the National Ignition Facility (NIF) in the United States.
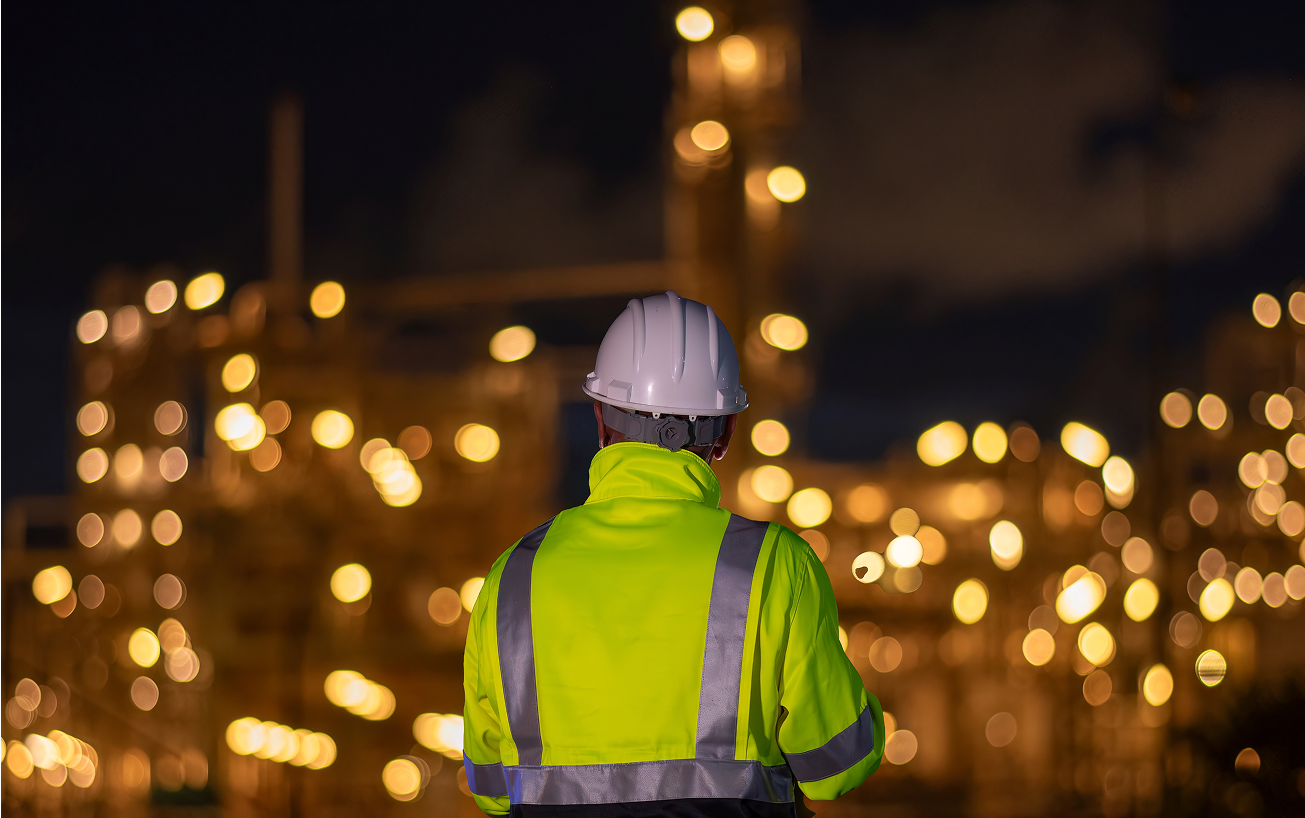
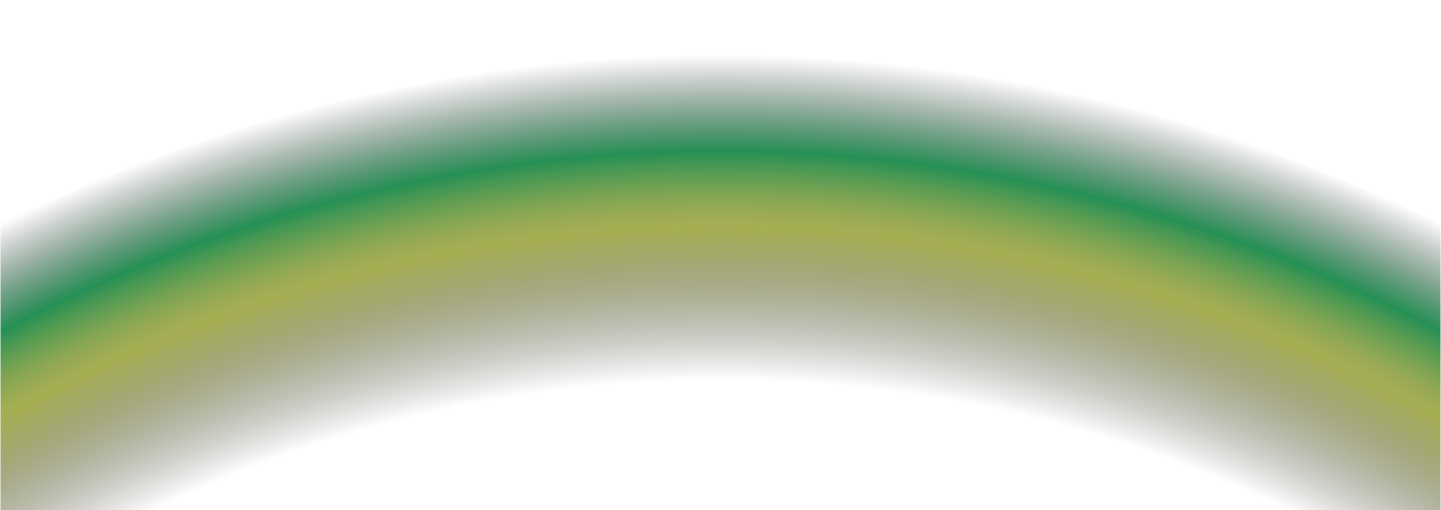
Contact us
Do you have any questions? Don’t hesitate to write to us.
We’re here to help!