Inertial confinement fusion
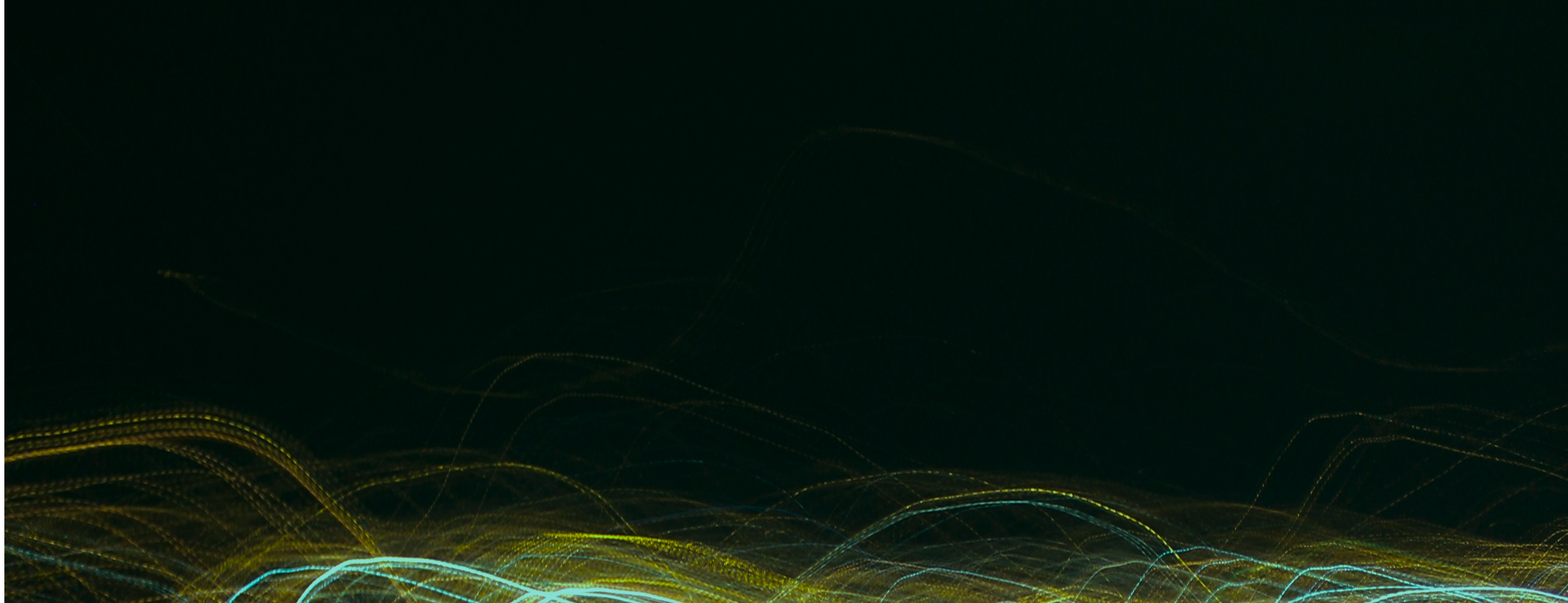
Inertial confinement fusion :
A path to carbon-free energy?
Scientists are trying to harness nuclear fusion reaction since the 1950s. Indeed, fusion of the two isotopes of hydrogen, Deuterium and Tritium (“DT”), generating an alpha particle and a neutron, can produce 337 MJ per mg of fuel, promising abundant, carbon-free energy. The scientific community is pursuing two approaches in this quest: magnetic confinement fusion (“MCF”) and inertial confinement fusion (“ICF”).
Hervé Besaucèle o GenF, Elancourt – France | herve.besaucele@genf-systems.com
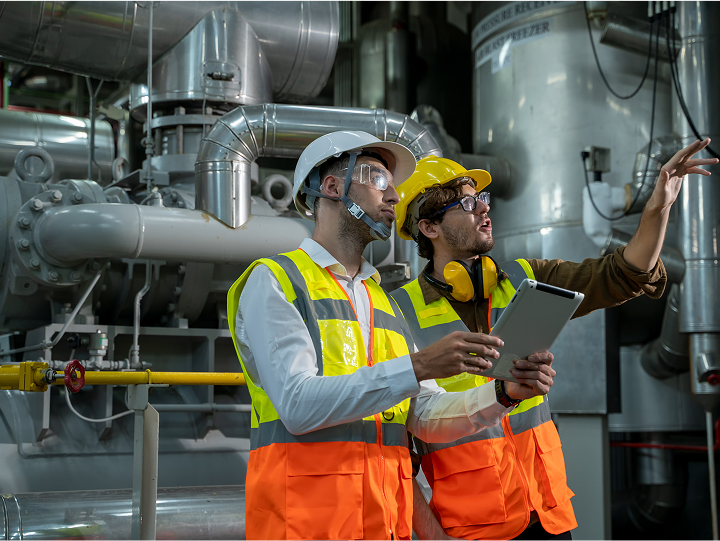
Scientists are trying to harness nuclear fusion reaction since the 1950s. Fusion of the two isotopes of hydrogen, Deuterium and Tritium (“DT”) can produce 337 MJ per mg of fuel, promising abundant, carbon-free energy. The scientific community is pursuing two approaches in this quest: magnetic confinement fusion (“MCF”) and inertial confinement fusion (“ICF”).
If MCF has taken the major part of attention and budget in fusion for energy, with the ITER project and other research and private initiatives, ICF has taken a significant step on December 5th, 2022 by being the first to reach the long thought after “ignition point”.
On this day, the National Ignition Facility (NIF) at the Lawrence Livermore National Laboratory (LLNL) in California generated 3.15 Megajoule (MJ) of nuclear energy by imploding a capsule filled with Deuterium and Tritium with 2.05 MJ of laser energy. The principle of ICF (fig 1) is to irradiate a millimetre-scale spherical capsule filled with deuterium and tritium with very high power radiation during a few nanoseconds. The ablation of the outer surface of the capsule leads to compression of the DT fuel, leading to density and temperature (~ 100 millions K) sufficient to trigger fusion reactions.
The alpha particles generated are then re-absorbed in the plasma, increasing further its temperature to trigger a large amount of fusion reaction that can consume a large fraction of the DT fuel. Reaching this state of burning plasma necessitates extremely symmetric irradiation and very tight capsule tolerance.
Name – Direction
This has made the quest for ignition elusive until the NIF breakthrough, repeated five times since 2022, with energy output reaching 5.2 MJ for 2.2 MJ of invested laser energy, confirming the maturation of the inertial confinement fusion (ICF) science. Those spectacular results are opening the door for finally making true the precept that fusion has always been “30 years away”. Therefore, a number of public and private initiatives are now underway to take on the significant technological and engineering challenges that stay in the road between ignition at NIF and viable carbon-free ICF based power plant.
From ignition to a fusion power plant?
In an extremely simplistic way, a fusion power plant will follow the basic principle highlighted by the schematic of Fig 2. A capsule filled with about one mg of deuterium and Tritium is injected in a reaction chamber where it will be irradiated by multiple laser beams of megajoule energy. The implosion of the capsule can lead to the combustion of up to 30% of the DT, leading to the release of more than 100 MJ. Repeating the process multiple times per second will result in Gigawatt average power generated mostly in the form of fast neutrons (14.3 MeV).
A lithium-based compound will absorb neutrons, providing two functions: convert the energy to heat and regenerate Tritium (whose supply is extremely scarce on earth). The conversion of heat to water vapour and electricity will then be very similar to a traditional power plant.
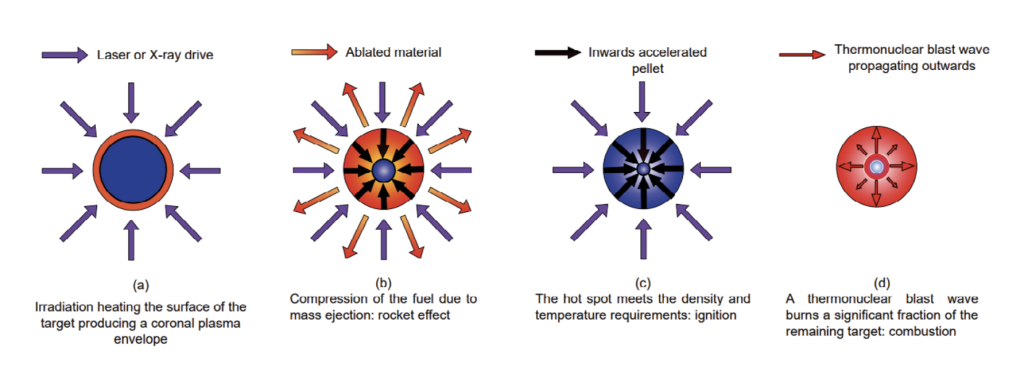
Figure 1. Principles of inertial confinement fusion (taken from Ref 3).
The path to large gain
The gain of the reaction (ratio of fusion energy divided by laser energy) has to be large enough to compensate the energy required for the lasers and other sub-systems in the power plant. This is not the case in NIF where a gain of ~ 2.5 does not come close to compensate the laser efficiency far below 1%. The experiments at NIF and its French equivalent the Laser Mega Joule (“LMJ”) use the so-called indirect drive irradiation.
The gain of the reaction (ratio of fusion energy divided by laser energy) has to be large enough to compensate the energy required for the lasers and other sub-systems in the power plant. This is not the case in NIF where a gain of ~ 2.5 does not come close to compensate the laser efficiency far below 1%. The experiments at NIF and its French equivalent the Laser Mega Joule (“LMJ”) use the so-called indirect drive irradiation.
In this case, X-ray radiation, generated by interaction of the main laser beams with the inner wall of a cylindrical cavity (“Holrhaum”), implodes the deuterium-tritium capsule. It benefits from the X-ray very short wavelength but has a poor efficiency, only a small fraction of the laser energy being coupled to the capsule. Even if NIF expects gains of around 15 with indirect drive in a near future, direct drive, where the laser beams directly irradiate the capsule seems more promising to reach gains of 100 or more. All potential ICF “recipes” face a major challenge: controlling the instabilities at work during the implosion. Hydrodynamic instabilities occur at the interface between two fluids moving in opposite direction (in case of ICF, the light and hot ablated matter against the heavier and cold capsule) or at different speeds (see Fig. 3). They will grow from any even minor non-uniformity in laser irradiation (“laser imprint”) or capsule structure, stopping the compression from converging towards ignition conditions.
Laser-plasma instabilities (“LPI”) exacerbate those non-uniformities and reduce efficient coupling of the lasers into the plasma. LPI include several non-linear processes occurring when a high-intensity laser interacts with a plasma:
- stimulated Brillouin scattering (“SBS”)
- stimulated Raman scattering (“SRS”)
- two-plasmon decay (“TPD”)
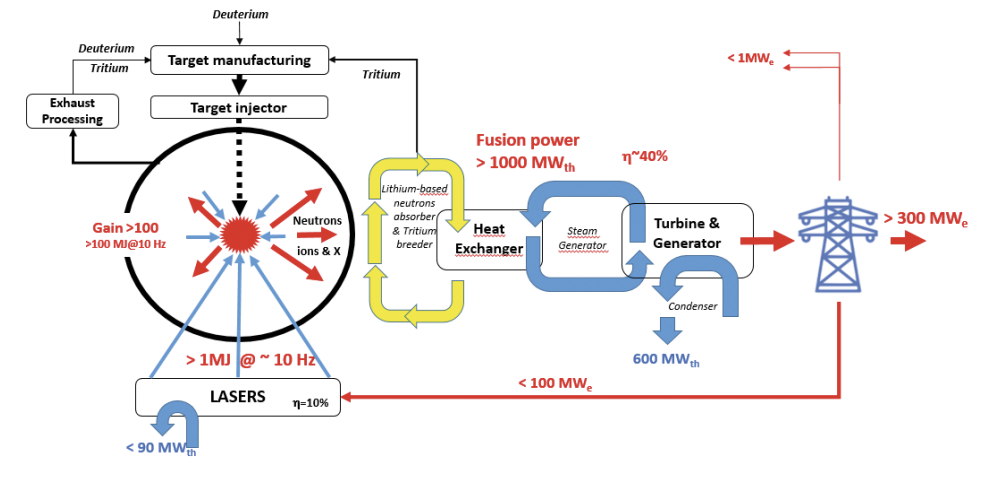
Figure 2. Simplified principle of an ICF power plant
References :
[1] H. Abu-Shawareb et al. Phys. Rev. Lett. 132, 065102 (2024) [2] R.E. Olson et al., Plasmas 28, 122704 (2021) [3] Diego Viala, “Study of the physics of shock ignition,” Physics. Université de Bordeaux, 2024.English.
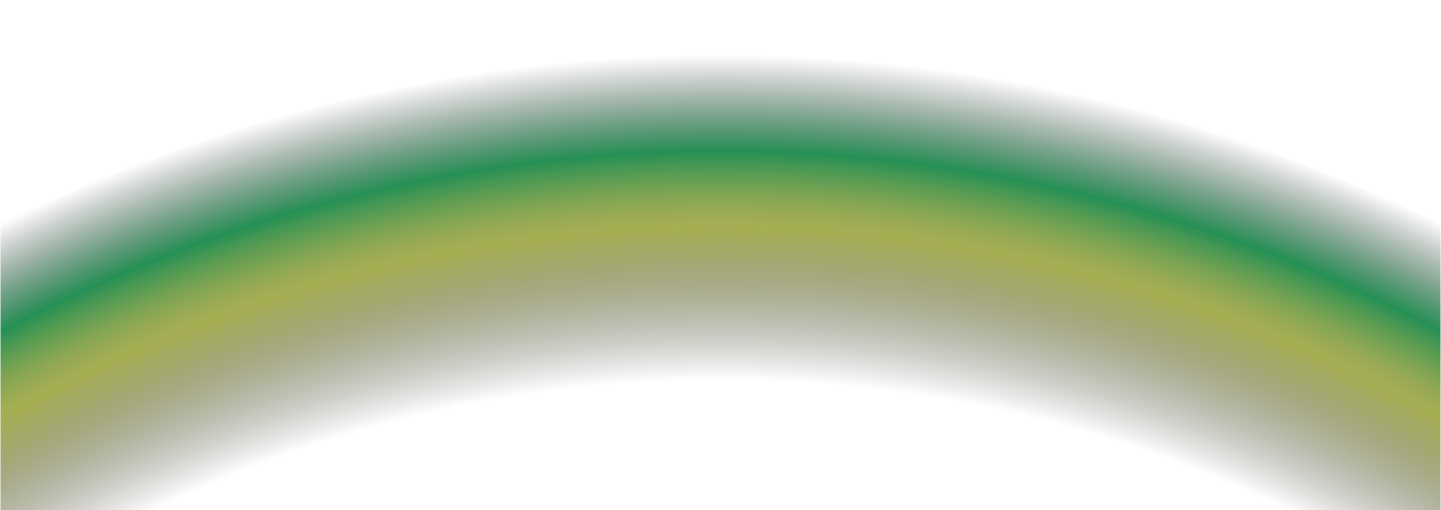
Contact us
Do you have any questions? Don’t hesitate to write to us.
We’re here to help!